Rockefeller cell cycle scientists test the use of ROTOR+ for Chlamydomonas Cell Culture
Introduction
Molecular tools like CRISPR gene editing have unleashed the potential of many more different organism types for genetic studies and potential industrial applications. Green algal researchers are among those leading the charge towards species diversification, having identified the eukaryotic alga Chlamydomonas reinhardtii (Chlamydomonas) as an ideal microbial genetic model for the plant superkingdom.
So, while the ROTOR+ high-throughput screening robot was initially developed for use in yeast (S. cerevisiae to be specific), it seems natural to ask, can it efficiently pick other organisms? However, Chlamydomonas differs from budding yeast in cell size, growth rate, colony morphology adhesiveness, and light responsiveness.
To find out how well ROTOR+ might perform with the gloopy morphology of green algae, Professor Fred Cross and his team decided to put the robot to the test.
“The yeast projects were decade long projects involving many, many labs. With the availability of these instruments to accelerate getting the mutants to begin with, combined with deep sequencing to identify the responsible mutant genes, we should be able to go a lot faster.”
Professor Fred Cross, Rockefeller University
Method
Manual replication plating
384 mutants per plate
= 10 mutants per experiment
Automated replication plating with ROTOR+
6,144 mutants per plate
= 1,000 mutants per experiment
Professor Cross and his team have come up with the ideal solution. By combining Singer Instruments’ ROTOR+ and PhenoBooth+ high density arraying and phenotyping systems, they have developed a high-throughput method of applying traditional genetic linkage analysis to scale their backcrossing experiments to nearly 1,000 mutants per experiment compared to only 10 mutants per experiment using manual methods (Kafri et al, 2023).
What’s more the approach achieves a false positive rate of less than 11% – up to five times lower than existing methods. This paves the way for scientists to identify and functionally characterise the genes required for photosynthesis with unprecedented confidence and scale, driving the discovery of previously hidden regulatory components and photosynthetic pathways.
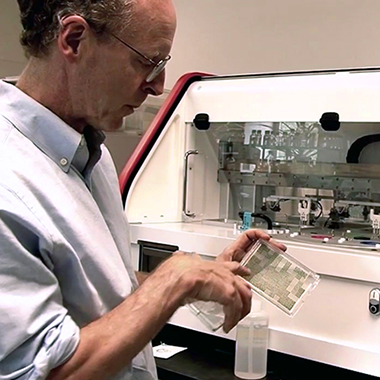
Results
Fred started with a 384 mutant library in liquid suspension.
Using ROTOR+ he successfully arrayed his culture up to 6144 density – in less than a day!
“Fortunately in every case the ROTOR+ seemed completely compatible with Chlamydomonas and we continue to find new uses for it.”
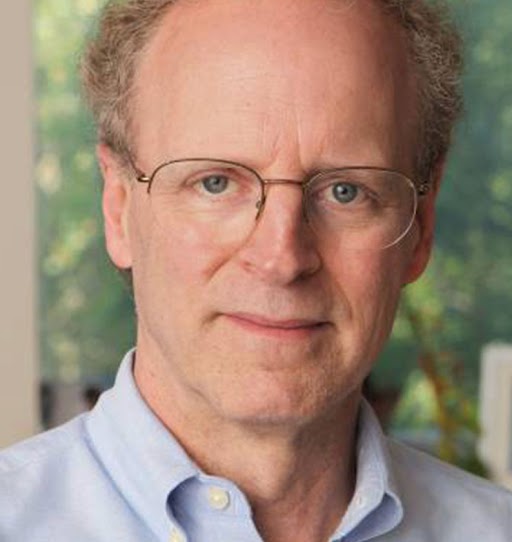
Professor Fred Cross
Rockefeller Laboratory of Cell Cycle Genetics
Get Fred’s full report
Professor Cross has been successfully employing Singer Instruments’ robots to run large-scale Chlamydomonas genetic screens since 2012.
Download his white paper for access to all the data from his personal analysis of ROTOR+’s accuracy and performance with C. reinhardtii.