From yeast genetics to the secret weapon of bioengineering
Professor Ed Louis shares how ROTOR+ has facilitated his pioneering work into yeast population genomics.
Studying genomic interactions in yeast
There are millions of possible different variant combinations within a given genome, making the study of genetic interactions extremely challenging. High throughput genetic screens capable of deducing such interplay were first pioneered in the early 2000’s, with the development of Synthetic Genetic Array (SGA), involving the analysis of thousands, if not millions of pairwise interactions at a time.
SGA led to groundbreaking work to systematically map all 36 million genetic interactions in budding yeast (Costanzo et al, 2016). It provided some early insights into how genetic interactions might map to complex human diseases (Son Sung et al, 2016) and paved the way for a wide variety of studies employing mutant allele collections for yeast functional genomics (Costanzo et al. 2019).
But it wasn’t the full picture. And it wasn’t long before many in the yeast community became curious about just where else all that remaining ‘missing heritability’ might be concealed (Manolio, 2009).
Among them was Professor Ed Louis, whose cornerstone Nature paper demonstrated the enormous variation found within domestic and wild yeasts and was one of the first large-scale efforts to apply high-throughput sequencing technology to S. cerevisiae (Liti et al, 2009). Crucially, it laid the foundations for more widespread interest in S. cerevisiae as a model for population-wide genomics unpicking complex traits, rapidly elevating it from laboratory lackey, to its status today as a driving force behind biological innovation (Gaikani et al, 2024).
Ed’s extensive knowledge of yeast population genomics led him to found Phenotypeca in 2018, employing proprietary Quantitative Trait Loci (QTL) technology to optimise for recombinant protein production across a variety of fields including vaccines, therapeutics and cosmeceuticals (Finnis et al, 2022). The iterative process involves screening billions of progeny, aiming to produce a near-optimal strain from the get go, ready for commercially scalable manufacture.
As a long-term customer of Singer Instruments and an early adopter of ROTOR+, Ed was more than happy to share his thoughts with us about the power of yeast for bioengineering. Our product specialist, Ailsa Stevens, caught up with Ed in the fall of 2024 to talk about the significance of ROTOR+ PIXL and how it has grown to become somewhat of a ‘secret weapon’ for the industry.
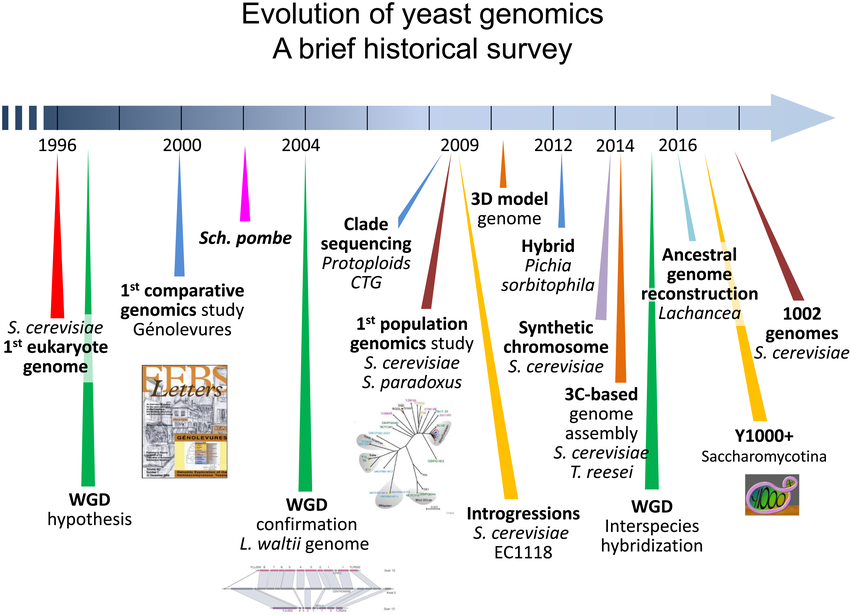
Major studies and discoveries in yeast genomics since the sequencing of Saccharomyces cerevisiae (see main text for details). S. pombe: Schizosaccharomyces pombe; S. paradoxus: Saccharomyces paradoxus; T. reesei: Trichoderma reesei; WGD: whole‐genome duplication; L. waltii: Lachancea waltii – Image source: https://www.researchgate.net/figure/Major-studies-and-discoveries-in-yeast-genomics-since-the-sequencing-of-Saccharomyces_fig2_330407622
ROTOR+ has been a workhouse of yeast genomics for more than two decades.
Read the full interview with Professor Ed Louis below.
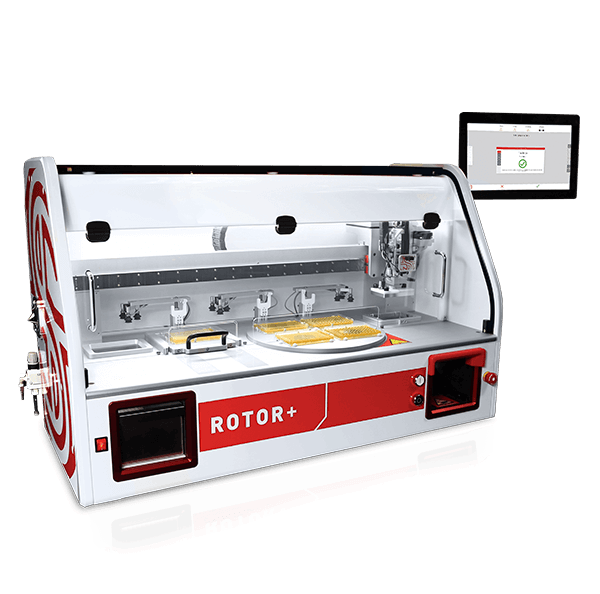
ROTOR+ was first created to automate SGA for the study of genetic interactions. How have things moved on since then?
“The lab strain of yeast is not actually very good for most traditional yeast uses. It doesn’t make very good bread or beer or wine, (even though we did put that to the test). However, it and its derivatives have been successfully used as a chassis for expressing heterologous proteins. And it’s been used in experiments leading to three Nobel prizes.
But it’s not actually a good representative of the genetic variation across the species as a whole, nor is it necessarily optimum for any really desirable phenotypes. That’s because it’s somewhat of a ‘mongrel’ created from lots of different crosses that give it properties useful for growing in the lab.
Because of this we have always been more interested in the vast genetic diversity found in the natural world. That meant we diverged quite early on from the groups doing SGA. And so for the 2009 Nature paper we had the sequences of these 35 or 36 different strains. About half were from wild populations, adapted to their niches and geographic locations. And then the other half we looked at were more or less shaped by human activity moving them around, allowing them to interbreed.
This really paved the way for lots of different functional genomics approaches involving crossing multiple strains simultaneously. We didn’t do just one generation, we did six, twelve, eighteen generations. It meant we had a lot more segregating variants, and their combinations, and we didn’t just focus on one pair of parents. We did multiple crosses to give us larger insights into more complex traits.
In fact, with one cross, I think there were 24 variants involved. We genotyped 960 combinations using the ROTOR+ and that revealed there were over 750 genetic solutions to the problem. These advances in Quantitative Trait Analysis (QTL) in yeast soon taught us that, when dealing with highly complex traits, you can’t just deal with just one genetic background.
And we achieved that using high density arrays of yeast on the ROTOR. It allowed us to ask completely novel questions like: out of a billion progeny, which million would continue to grow at 40 degrees. And that work led us to different combinations of genetic variants that were responsible for giving heat resistance.“
How did all this foundational work in yeast population genomics lead you to found Phenotypeca?
“Phenotypeca was founded based on this knowledge that there’s an absolutely huge amount of hidden genetic diversity out there. And essentially beneficial variants are only expressed in the right genetic background.
So this is where many biotech and biopharma companies are essentially barking up the wrong tree. They are trying to screen different yeast strains, to find better ones and then throw out the bad ones. But what we’re finding is that the bad ones generally contain really beneficial variance hidden in them, and you’re not going to find it without doing in-depth QTL.
Our approach at Phenotypeca is to run through a whole series of tests, with the goal of creating highly customised and patent protected strains. The primary aim is to produce commercially viable levels of a product in strains that don’t require as much downstream processing. We have multiple diverse libraries, and each potential product will be matched with a library it is anticipated to perform well in. Successive iterations of breeding lead to much more highly optimised strains and a premium end product.
The numbers are big. A typical cross for us might have 100,000 SNPs. And even if we only deal with two parents, that’s equivalent to 1030,000 different potential genotypes. There are only 1081 atoms in the universe. So effectively we’re dealing with thousands of progeny at a time, or a billion if we have a pooled selection of strains rather than a screen. We’re aiming to optimise not just for titre but reduced downstream processing to have a high-quality lower cost product at the end.“
How are ROTOR+ PIXL helping to meet the demands of Phenotypeca’s growing customer base?
“ROTOR+ PIXL are crucial to our approach as we could never keep up with that level of iteration without them. They are really the workhorses behind the whole operation. The lab automation we need is not generic off the shelf stuff, it’s highly specialised. And these two instruments just fit exactly what we need them to do, with the necessary flexibility to scale, both in size of project and numbers of projects.
Certainly for us having ROTOR+ is very significant. Those in the team that have prior experience with it from their academic careers will know that we are far ahead of the game with ROTOR+ in-house.“
Looking for screening tools to accelerate your research?
ROTOR+: the only microbial screening solution capable
of pinning a million colonies an hour.